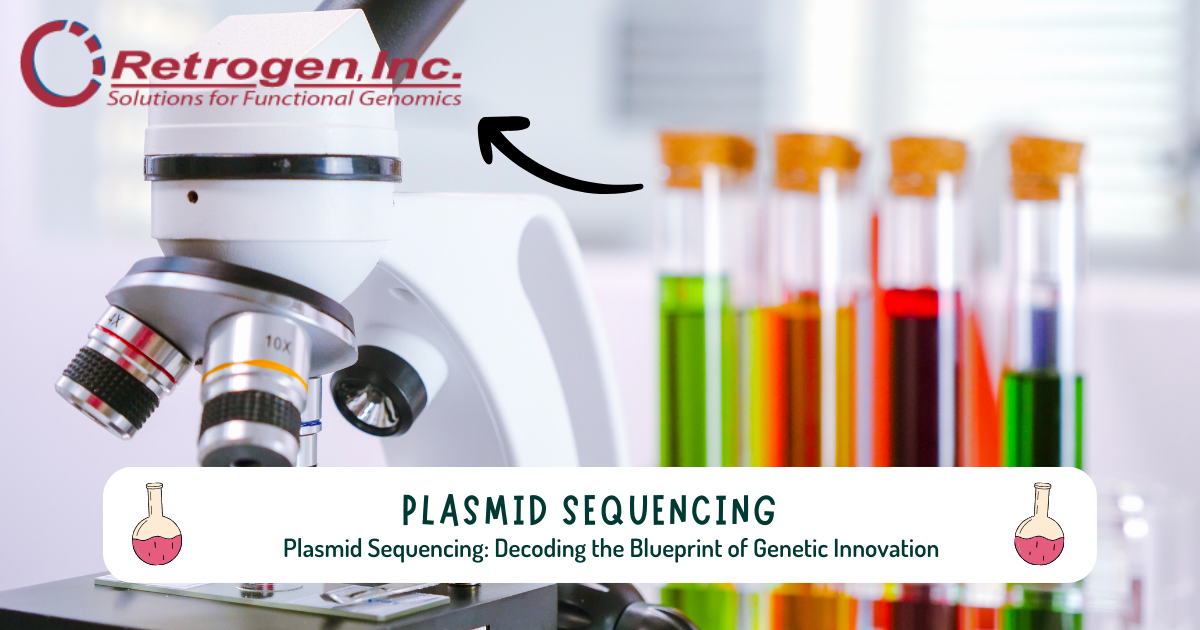
Within the ever-evolving domain of hereditary qualities, Plasmid Sequencing stands as a foundation for groundbreaking disclosures. This article digs into the subtleties of plasmid sequencing, unraveling its importance and applications in present-day hereditary investigation.
Plasmid Sequencing Unveiled: Understanding the Basics
Set out on a travel through the elemental standards of plasmid sequencing. From DNA extraction to intensification, get a handle on the basic steps that clear the way for unraveling hereditary puzzles.
Navigating the Genetic Code: The Role of Plasmid Sequencing in Research
Explore how plasmid sequencing acts as a compass in decoding the intricate genetic code. Learn how researchers leverage this technique to unlock valuable insights into genetic variations, enabling advancements in various fields.
Revolutionizing Medicine: Plasmid Sequencing in Therapeutic Innovations
Delve into the revolutionary impact of plasmid sequencing on medical breakthroughs. Uncover how this technique is pivotal in developing innovative therapies and treatments, offering hope for numerous genetic disorders.
Troubleshooting in Plasmid Sequencing: Overcoming Challenges
Navigate through common challenges encountered in plasmid sequencing. Gain insights into troubleshooting techniques, ensuring a smoother journey in decoding genetic information accurately and efficiently.
Plasmid Sequencing Techniques: From Sanger to Next-Gen
Dive into the evolution of plasmid sequencing techniques. Compare traditional Sanger sequencing with cutting-edge Next-Generation Sequencing (NGS), understanding the advantages and limitations of each method.
FAQs: Answering Your Queries on Plasmid Sequencing
What is the significance of plasmid sequencing in genetic research?
Plasmid sequencing plays a crucial role in deciphering specific genetic traits, aiding researchers in understanding the intricacies of DNA, and facilitating advancements in genetic therapies.
How does Next-Generation Sequencing differ from traditional methods in plasmid sequencing?
Next-generation sequencing allows for high-throughput, rapid sequencing, surpassing the limitations of traditional methods like Sanger sequencing, thus accelerating genetic research.
Can plasmid sequencing be applied in agriculture?
Absolutely. Plasmid sequencing finds applications in modifying crops for enhanced resistance, nutritional value, and overall agricultural sustainability.
Is plasmid sequencing accessible for independent researchers?
With technological advancements, plasmid sequencing has become more accessible and cost-effective, empowering independent researchers to contribute to genetic exploration.
What challenges do researchers face in plasmid sequencing, and how can they be addressed?
Common challenges include contamination and data analysis complexities-rigorous laboratory practices and advanced bioinformatics tools aid in overcoming these hurdles.
Conclusion: Embracing the Future of Genetic Discovery As we navigate the intricate realm of plasmid sequencing, it’s evident that this technique is not just a tool; it’s a gateway to a future where genetic discoveries hold the key to transformative advancements. Stay curious, stay informed, and unlock the potential of genetic innovation.